Research
Emergent electronic phases in van der Waals heterostructures
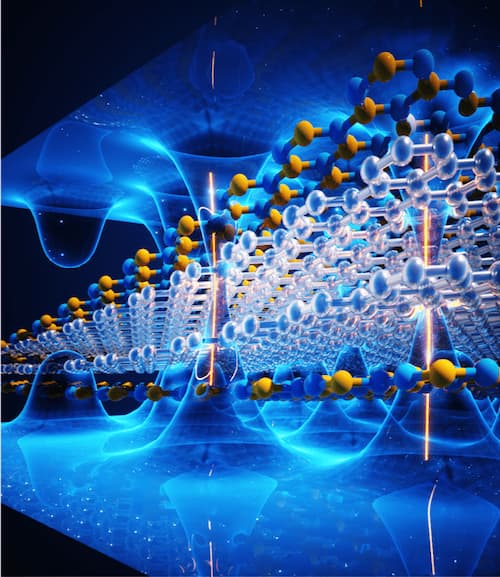
The concept of emergence is at the heart of the condensed matter: Although all materials are composed of atoms and electrons, these particles can organize in various ways, giving rise to emergent phases and phenomena that can be dramatically rich and cannot be understood by studying the individual constituents. The emergent quantum phases, however, depend sensitively on the charge carrier density, symmetry, electron-electron interactions, and other material parameters. How we can precisely control those parameters to realize new quantum emergence becomes a great challenge.
Two-dimensional vdW materials provide an extremely powerful material platform to design and study emergence. Specifically, their chemical potential can be controlled by electrostatic gating. Their symmetry and electronic structures can be designed by layer stacking. They can be coupled with superconductors/ferromagnets for proximity effects. They can be integrated into cavity/plasmonic structures for enhanced light-matter interaction. Particularly, they can form moiré superlattices through interference between two mismatched atomic layers. Moiré superlattices can host a series of emergent phases, including correlated insulators, superconductors, topological magnets, and ferroelectricity. Our group is interested in (1) realizing new moiré materials and new emergent phases; (2) developing new experimental tools to control and probe those materials and phases.
For instance, we recently uncovered peculiar electrical switching of resistance and electronic ratcheting effect in graphene-boron nitride moiré superlattices:
Unconventional ferroelectricity in moiré heterostructures, Nature 588, 71 (2020)
Electronic ratchet effect in a moiré system: signatures of excitonic ferroelectricity, arXiv: 2306.03922 (2023)
Related readings from others:
Electrical switching of a bistable moiré superconductor, Nature Nanotechnology 18, 331–335 (2023).
Ferroelectricity in hBN intercalated double-layer graphene, Frontiers of Physics 17, 43504 (2022).
Giant ferroelectric polarization in a bilayer graphene heterostructure, Nature Communications 13, 6241 (2022)
Moiré synaptic transistor for homogeneous architecture reservoir computing, Chinese Phys. Lett. 40, 117201 (2023).
Condensed Matter Journal Club commentary: “The Curious Case of the Gate that Doesn’t Work” Recommended with a Commentary by Brian Skinner (Ohio State University) and Matthew Yankowitz (University of Washington)
Electromagnetic responses and quantum geometry

The concept of geometry plays vital roles in multiple disciplines, ranging from mathematics, cosmology, and physics, to arts. For instance, geometry lies at the heart of space-time. In free space, light travels in straight lines. However, close to large masses, the geometry of space-time warps, bending the trajectory of light. In quantum materials, quasiparticles are also constrained by an emergent quantum geometry, which originates from the local geometrical structure of electron quantum wavefunctions. Prominent quantum geometrical properties include quantum metric, Berry curvature and Berry connection. These quantum geometrical properties capture the coherences between orbitals, atomic positions, and spins within the unit cells, which have deep connections with the topological properties of the materials.
Similar to how the geometry of space-time can drastically bend the light trajectory, these quantum geometrical properties in materials can strongly modify the electromagnetic responses of materials. Our group builds unique capabilities combining electrical and optical approaches to detect electromagnetic responses over a wide spectrum (from near DC, THz, infrared to UV) to search for new quantum geometrical responses. In turn, these responses will allow us to directly probe Berry curvature, Berry phase, and topological properties of a wide range of quantum materials.
For instance, our recent work demonstrates an exotic chiral electronic phase that emerges through light-matter interactions:
Spontaneous gyrotropic electronic order in a transition-metal dichalcogenide, Nature 578, 545-579 (2020) (Cover story)
Quantum metric nonlinear Hall effect in a topological antiferromagnetic heterostructure, Science 381, 181-186 (2023)
Emergent phases and quantum geometry under dynamical drive
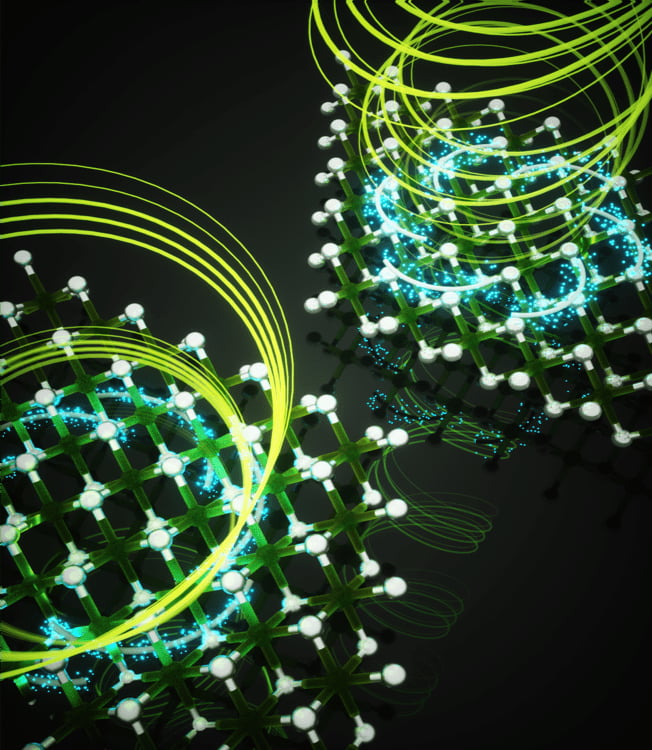
New electronic and quantum geometrical phases can emerge when the material is driven by light. The light can pump quasiparticles, dynamically break symmetry and dress electronic states, which can lead to new electronic phases. For instance, photon-dressing can turn a topologically-trivial material into a topological insulator. People have observed light-induced superconductivity in non-superconductors under equilibrium. It has also been recently predicted that optical pumping may make the moiré bands even flatter, leading to light-enhanced electron correlations. These phases represent the crossroads of topology, correlation, and quantum dynamics. These new phases expect to exhibit rich quantum geometrical properties and novel electromagnetic responses. Our group is interested in discovering new phases through light-matter interactions and studying their dynamics.
For instance, we recently uncovered that second-order anomalous Hall effect can arise from either Berry curvature dipole or quantum metric dipole:
Observation of the nonlinear Hall effect under time reversal symmetric conditions, Nature 565, 337–342 (2019)
Quantum metric nonlinear Hall effect in a topological antiferromagnetic heterostructure, Science 381, 181-186 (2023)
Also, two relevant review articles:
Topology and geometry under the nonlinear electromagnetic spotlight”, Nature Materials 20, 1601–1614 (2021)
Photocurrent as a multi-physics diagnostic of quantum materials, Nature Reviews Physics 5, 170–184 (2023)
Welcome to Join Us!
“Synthesizing” new materials, building electronic and optical devices, developing new mechanics and electronics to control the fine parameters of the materials.
Join us to play with lasers and optics, and detect mechanical and electronic properties.