Photographs by Gary Wayne Gilbert
In early May 1985, an 11-month-old girl named Janice was crawling around the kitchen in her grandmother’s Brooklyn brownstone. The older woman was making pasta, and as she carried the pot to the sink to drain the boiling water Janice scooted underfoot. The woman tripped, spilling the searingly hot liquid over her granddaughter.
An ambulance rushed Janice to New York Hospital, on the east side of Manhattan, where Kevin Tracey, a surgical resident, was on duty. “When I met Janice, she was scalded over her entire body,” Tracey recalls. “It was awful.”
For the next few weeks, Tracey was part of the medical team that cared for Janice, giving her painkillers, changing her dressings, and shuttling her to the operating room for occasional skin grafts. Slowly, Janice began to recover, and her doctors started making plans to send her home. In late May, the hospital staff threw Janice a party, complete with cake and streamers, to celebrate her first birthday.
The next day, a nurse was feeding Janice from a bottle when the girl’s eyes suddenly rolled back and her heart stopped beating. Tracey, who happened to be passing in the hall, rushed into Janice’s room and began giving her mouth-to-mouth resuscitation. A more senior doctor soon arrived to assist, and for more than an hour they tried to revive the toddler. But it was no use: Janice was gone.
It fell to Tracey to give Janice’s parents the terrible news, a job made harder by the fact that he couldn’t explain why their daughter, who seemed to be on the mend, had died so suddenly. The medical team believed that Janice had succumbed to septic shock, a rapid drop in blood pressure triggered by an infection, but when they examined her body they couldn’t find the microbe that might have caused it.
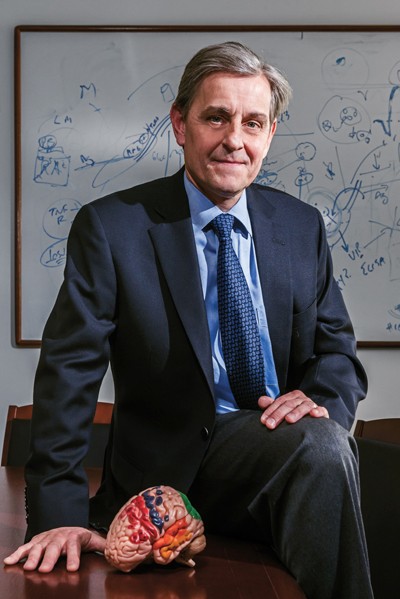
Janice’s death was a tragedy, but not an anomaly: At the time, much remained unknown about septic shock, and even in patients who ultimately died from the condition, doctors were sometimes unable to identify the microbial culprit. Tracey had always felt driven to understand precisely how things worked—he had been the kind of kid who liked to take apart cars—and he felt a desperate need to solve this mystery. “Here this little girl had died in this tragic way, and I didn’t know why,” he recalls. “And I said, well, you know, we gotta figure this out.”
Within weeks of Janice’s death, Tracey was in the laboratory, studying the biological mechanisms behind septic shock. That single project would send the neurosurgeon-in-training on a 30-year detour into the field of immunology, ultimately yielding a surprising discovery—published in Nature and described in Scientific American and New York Times Magazine cover stories—about the links between the immune system and the nervous system. Tracey’s unexpected finding—that a nerve originating in the brainstem modulates the body’s immune response—has helped lay the foundation for the nascent field of bioelectronic medicine, in which diseases are treated not with pills but with electrodes. If the clinical trials now underway continue to pan out, patients with rheumatoid arthritis, inflammatory bowel disease, psoriasis, and other inflammatory disorders may one day be able to use implantable, nerve-stimulating devices to keep their symptoms at bay. “I went into the laboratory to study the immune basis of sepsis in 1985,” says Tracey, who is now the president and CEO of the Feinstein Institute for Medical Research in Manhasset, New York. “And you could say that in many ways I’ve never come out.”
The Feinstein Institute is housed in a gleaming, glass-and-brick building just off the Long Island Expressway, part of a small medical campus sandwiched between several country clubs. The Institute is the independent research division of Northwell Health (formerly known as the North Shore–LIJ Health System), the largest healthcare provider in New York State. Its more than 1,500 researchers conduct laboratory and clinical studies into the treatment and prevention of human disease. Tracey, who runs the Laboratory of Biomedical Science, has helmed the Feinstein Institute, which has a current budget of $135 million, since 2005. He works from a corner office on the fourth floor, with large windows that overlook a tree-lined road. The trees are still green on a drizzly, gray day in late November when he sits down for a chat at the small conference table in his office.
Tracey is an attentive and focused listener, but even when he is sitting he rarely comes to a complete stop. As he speaks about his life in the lab, he rocks back and forth in his chair. He runs his fingers through his silver hair. He adjusts his tie. He drags his hands across the tabletop, drawing invisible diagrams. He hops up to pull a book off a shelf, to find a photo of a patient, to grab a sample of an experimental drug he helped create. This buzzing, restless energy is fitting for a man who talks easily, and often, about discovering his purpose when he was barely old enough to read. “What I’ve always wanted to do, for my whole life, is run a laboratory and invent things and try to discover things that would help people,” he says. “That’s been the guiding principle of my entire life.”
The oldest of five children, Tracey spent his early years in Western Massachusetts. In the summer of 1963, when he was five years old, his mother began getting headaches. Her local doctor referred her to a specialist at Yale, so Tracey and his siblings went to stay with their grandparents while their parents headed to New Haven. Tracey never saw his mother again; within weeks, she had died of a malignant brain tumor.
One day, shortly after his mother’s funeral, Tracey climbed into his grandfather’s lap, wanting to know why the doctors hadn’t been able to save his mother. His grandfather, a doctor himself, explained that her tumor was like a crab, with legs that extended into so many different parts of her brain that it had been impossible to remove safely. “And so at that very young age, I remember saying to him, ‘Well somebody should do something about that,'” recalls Tracey. He decided that he would become that somebody: a doctor or a scientist who discovered new treatments for the sick. “I remember wanting to invent things to help other kids’ moms so other kids wouldn’t have to go through what I went through.”

When he arrived at Boston College, in 1975, he finally got the chance to see what that dream might look like. On his first day of organic chemistry, Tracey watched, awestruck, as professor T. Ross Kelly stood at the board, drawing diagrams of molecules that he was synthesizing in his lab. The compounds, Kelly told the class, were potential treatments for cancer. “I almost fell out of my chair,” Tracey recalls. “I thought to myself, ‘All my life I’ve wanted to use science to help people, and here my teacher, whose laboratory is 100 yards away from this lecture hall, is building molecules for cancer patients.'”
At the end of the semester, Tracey took a job as a research assistant in Kelly’s laboratory, where he quickly impressed his professor. “He was full of energy, full of enthusiasm, and smart,” Kelly recalls. “That’s a great combination.”
The two years that Tracey spent in Kelly’s lab convinced him that he was on the right path. “I saw the research process,” Tracey says. “I saw invention happen . . . and I knew that I wanted to be a part of that.”
And so, Tracey enrolled in medical school at Boston University in 1979 knowing that, in addition to being a clinician, he wanted to carve out time to spend in a laboratory. He was just a few months away from beginning a long-planned two-year research fellowship—on a subject yet to be decided—when Janice arrived in the Manhattan ER in the spring of 1985. That July, he joined the lab run by Stephen Lowry, a surgeon and scientist at Cornell University Medical College, and began to study the cellular underpinnings of septic shock. Working in collaboration with several other scientists—including Anthony Cerami and Bruce Beutler at Rockefeller University—Tracey made two major findings in quick succession. First, he demonstrated that in at least some cases of septic shock, patients were killed not by invading bacteria, but by their own immune systems. When pathogens enter the body, white blood cells produce a compound called tumor necrosis factor, or TNF, an immune-signaling molecule that helps coordinate the destruction of trespassing microbes and triggers inflammation, which is part of a healthy immune response.
But high doses of TNF, Tracey found, caused collateral damage, injuring a patient’s own cells and tissues. When Tracey infused large amounts of TNF into healthy rats, the rodents rapidly went into shock, developed organ damage, and died. “The similarities to Janice’s case of shock were astounding—the onset of shock [was] sudden and devastating, and in both cases no bacteria were implicated,” Tracey wrote some 20 years later in a medical memoir titled Fatal Sequence: The Killer Within (Dana Press, 2005). “The conclusion was inescapable; all you needed to develop acute shock like Janice’s was too much TNF.” Perhaps, he speculated, even a minor, undetectable infection could set off this oversized immune response, triggering a sudden flood of TNF and rapid-onset shock.
Tracey published his findings in Science in 1986; the following year, he and his colleagues announced in Nature that they could protect baboons from septic shock by giving them a certain type of lab-made protein, known as a monoclonal antibody, that inhibited TNF. Together, Tracey’s experiments paved the way for a new suite of medical therapies. The overproduction of TNF is a feature of many inflammatory disorders—including rheumatoid arthritis, psoriasis, and inflammatory bowel disease—and TNF-blocking antibodies are now routinely prescribed for these conditions.

As Tracey completed his training in neurosurgery, he continued to research TNF. In 1992, after finishing his medical residency, he joined North Shore University Hospital in Manhasset, Long Island, where he finally got the chance to run his own lab. (Several years later, the hospital became one of the foundational facilities in the new North Shore–LIJ Health System, which established the Feinstein Institute as a standalone research organization.)
Tracey spent one day a week in the operating room—he specialized in spinal surgery—and the other four in his lab, where he searched for new ways to halt TNF rampages in the body. He developed one particularly promising drug candidate: a molecule he called CNI-1493, which blocked TNF production and reduced inflammation in animal models of several diseases, including sepsis, arthritis, and inflammatory bowel disease. Tracey thought the compound might prove similarly promising in treating stroke; data suggests that TNF overproduction during a stroke may worsen the resulting brain damage.
To test the idea, Tracey injected a small dose of CNI-1493 into the brains of rats. As he expected, the molecule blocked TNF production in the brain. But it also reduced TNF levels throughout the rest of the body. “This was a huge surprise,” Tracey recalls. “There was no way to understand—how could the molecule in the brain be turning off the TNF in the spleen, in the heart, in the liver?”
The finding was so unexpected that Tracey and his lab members puzzled over it for months, scouring the scientific literature for possible explanations. They didn’t find much. “The nervous system and the immune system had separate textbooks,” Tracey recalls. “They were not studied together.”
But if anyone could bridge the disciplinary gap, it was Tracey, a practicing neurosurgeon who had developed an unusual expertise in immunology. At first, he thought the brain might be sending messages about the CNI-1493 injection to the body through the bloodstream, but some simple experiments eliminated that hypothesis. He then began to consider the possibility that the brain was getting the word out via a different pathway: nerves.
He unearthed a crucial paper by Linda Watkins, a neuroscientist at the University of Colorado, Boulder, whose work suggested that compounds produced by white blood cells alert the brain to an infection by sending signals up the vagus nerve, which winds from the brainstem through the abdomen and regulates a variety of vital physiological phenomena, including respiration, digestion, and heart rate. And if immunological information traveled up the vagus nerve to the brain, Tracey reasoned, perhaps it also traveled in the opposite direction.
Tracey’s notion—that electrical pulses moving down the vagus nerve from the brain somehow turned off TNF production in the body’s other organs—was not wildly popular; in fact, several members of his own lab bet against it. To test the idea, Tracey repeated his previous experiment, injecting CNI-1493 into rats’ brains. But this time, he severed the vagus nerve. Although TNF levels once again plummeted in the brain, in the rodents’ other organs, they remained steady. The connection had been lost, and the messages weren’t getting through. (As for his winnings from the bet, Tracey says, “I think it was probably $20, and it probably never got paid.”)
Because nerves communicate by means of electrical signals, the finding raised the possibility that Tracey could dampen TNF production without any drugs at all. He borrowed a handheld nerve stimulator from one of the hospital’s operating rooms and used it to tickle the vagus nerve in several rats. The stimulation suppressed TNF production in the liver and reduced the amount of TNF circulating in the blood.
The discovery, published in Nature in 2000, suggested that the brain acted as a brake on the immune system, and led Tracey to propose what he called “the inflammatory reflex.” During an infection, he theorized, information about the body’s immune response zips up the vagus nerve to the brain. The brain then helps to calibrate this response by sending signals, via the vagus nerve, that curb the production of inflammatory molecules such as TNF. When it works properly, the circuit helps prevent the immune system from spiraling out of control and damaging the body’s own cells and tissues.
Given the dangers that inflammation can pose, it makes sense that this sort of self-regulatory pathway would evolve, says Alejandro Aballay, an immunologist at Duke University, who has identified specific neurons that control the immune response in roundworms. “It’s surprising that it has taken this long for the scientific community to appreciate this neural-immune connection.”

The discovery of the immune reflex immediately raised intriguing therapeutic possibilities. Although TNF-inhibiting drugs provide relief to many patients with inflammatory diseases, these medications have drawbacks: They are expensive, they have potentially serious side effects, and they don’t work for all patients. In 1997, shortly before Tracey performed his critical experiments, the U.S. Food and Drug Administration had approved a surgically implanted vagus-nerve stimulator as a treatment for epilepsy. Tracey began to wonder if a similar device might also function as a sort of pacemaker for the immune system, alleviating the symptoms of inflammatory diseases. While eating at a steakhouse, he jotted the idea down on a napkin (since gone missing): “Make a device to control the nerve to turn off TNF.”
In 2007, Tracey and one of his frequent collaborators, H. Shaw Warren, an inflammation specialist at Massachusetts General Hospital, founded SetPoint Medical to turn this back-of-the-napkin idea into a real-world medical treatment. The company partnered with Paul-Peter Tak, a rheumatologist at the Academic Medical Center in Amsterdam, to design and launch its first human trial, recruiting eight European patients with severe rheumatoid arthritis, an autoimmune disease in which high levels of TNF and related molecules cause inflammation in the joints. The disease can make the most mundane daily tasks difficult; some of the patients who enrolled in the trial had so much pain and swelling in their joints that they couldn’t button their own shirts, or use eating utensils, or stand up from a chair.
Tak and his colleagues repurposed the vagus-nerve stimulator approved for epilepsy, implanting a small, round “pulse generator” in each patient’s chest. For up to four minutes every day, the implant would send electrical pulses through a thin wire to the vagus nerve.
A middle-aged Bosnian man was the first to receive the implant, in the fall of 2011. A few months later, Tracey flew to Bosnia to meet him. There, the man told Tracey that before receiving the nerve stimulator the pain in his hands, wrists, elbows, knees, and feet had left him unable to work or play with his kids. But after the implant, he said, his pain had faded. He returned to his job as a truck driver. He took up ping pong again and enjoyed it so much that he began to play tennis, energetically enough to injure his knee. “So his doctors told him to take it easy,” Tracey says, laughing. “This is advice to a guy who had been on bed rest. Now they’re telling him to take it easy until the trial’s over.”
“Every time I think of that trip, I smile,” Tracey adds. “Seeing the joy on this patient’s face was a way to completely re-energize me and everyone else involved in working on extending this therapy to millions of people, not just one.”
Indeed, the Bosnian man was not the only one who seemed to benefit from the experimental treatment. At the annual meeting of the American College of Rheumatology in November 2012, the researchers announced that of their eight patients, six had a good or moderate response to the therapy; two of those six patients saw their arthritis go into complete remission. Overall, the group displayed significant improvement on a standard assessment of disease severity, after five weeks of daily nerve stimulation. Side effects, including swelling and numbness at the surgical site, were mild and temporary, according to Anthony Arnold, SetPoint’s CEO. Tak and SetPoint have now expanded the trial to an additional 10 patients; the results are currently being prepared for publication, but “those patients did really well,” Arnold says.
“Of course, this is still early days,” Tak acknowledges. The trial was small and did not include a placebo group for comparison. Not all patients responded to the treatment—not all patients respond to the standard drug regimen either—and Tak says that scientists still have a lot to understand about how to optimize this sort of treatment: Where is the best place to implant a vagus-nerve stimulating device? How long should the stimulation ideally last? But if researchers can answer these questions, and reproduce the effect in a larger, controlled clinical trial, “then I think it’s a very interesting approach for patients,” says Tak, who now heads immuno-inflammation research and development at GlaxoSmithKline, which has invested in SetPoint. “I think it’s a new paradigm that will really change the way we look at disease, and how we interfere with the natural resources in the body to dampen the immune response.”
Theoretically, the approach could have many advantages: Undergoing a minor surgical procedure to receive a device that automatically delivers pulses may be easier for patients than sticking with a daily drug regimen, and in the long run the implants may be cheaper. What’s more, drugs that block TNF can also have dangerous, immune-suppressing effects that leave patients vulnerable to infection and illness; it’s not yet clear what the long-term immunological effects of vagus-nerve stimulators will be, but some animal studies suggest that they may pose less risk.
SetPoint, which is developing its own proprietary vagus-nerve stimulator, is now sponsoring a clinical trial to test the treatment in patients with Crohn’s disease. And the promise of bioelectronic therapy extends beyond inflammatory disorders. Tracey predicts that scientists will ultimately identify nerves and neural circuits that are relevant to a wide variety of diseases. He says he wouldn’t be surprised to see clinical trials of nerve stimulators as potential treatments for multiple sclerosis, Alzheimer’s disease, fibromyalgia, hypertension, or even cancer. “I fully expect, based on the mechanistic knowledge we have today, that patients of the future will benefit from this,” he says. “How many patients and which diseases and what type of device? I’m not sure. But the scientific evidence indicates that this is a path forward.”
Despite these unanswered questions, Tracey’s scientific contributions have already been profound, says Carl Nathan, an immunologist at Weill Cornell Medical College. “I think it’s truly extraordinary that a practicing neurosurgeon has managed to discover so much new fundamental biology, including immunobiology.” Although Tracey’s work has sometimes challenged scientific dogma, his experiments are careful and convincing, Nathan says. “He’s passionate, generous, devoted to the people whose medical care he’s entrusted with, insatiably curious and remarkably creative,” he adds. “All of those qualities have driven him so far out in front of everybody that he’s kind of lonely there, and it may take a long time before [more] people realize the impact of what he’s discovered.”
Perhaps to speed the arrival of that moment, Tracey is in the process of launching a new Center for Bioelectronic Medicine, which he hopes will ultimately house 40 or 50 laboratories, at the Feinstein Institute. And in 2014, he started the journal Bioelectronic Medicine, as a means of connecting researchers from disciplines such as bioinformatics, bioengineering, neurophysiology, and nanotechnology.

Tracey routinely rises at 4:30 a.m. He tries to squeeze in a daily morning workout, and a bit of reading and writing, before departing the Greenwich, Connecticut, house he shares with his wife, Patricia McArdle Tracey ’83, and their two teenage daughters. (The couple also have two older daughters, who are in college.) He’s at the Feinstein Institute by 7:30 and spends the next 11—or more—hours juggling a variety of scientific and administrative tasks. He runs lab meetings and writes papers, applies for grants and gives talks, attends board meetings and solicits donations.
As a leader, Tracey says he has tried to create an environment in which researchers affiliated with the Feinstein Institute feel free to pursue unconventional ideas and take chances, even if not all their hunches pan out. “In order to encourage creativity in a research institution, you have to give people the ability to fail,” he says. “Which means that they know the resources are there so that if they fail they’re not out of work or their lab isn’t going to get closed.” And beyond simply providing resources, he adds, “You have to build a culture of trust—where people taking the risks know that other people around them are taking risks and that I take risks.”
Tracey no longer fixes spines or severs nerves with his own hands, but he still oversees the busy laboratory down the hall from his executive office, where a team of scientists is continuing to unpack the physiology of the inflammatory reflex. He helps design studies, and he reviews the data that streams in. “Nothing makes me happier than to be interrupted, even right now, by a student or a postdoc or a colleague to show me a result that, you know, is a happy surprise or an unexpected turn,” he says.
Although the lab is home to about 20 researchers, it’s quiet—almost deserted—this morning. But as Tracey wanders down the row of cluttered lab benches, he spies a Ph.D. student hunched over his computer. On the screen is an electrophysiological recording, featuring the telltale spikes of a firing nerve cell. The recording, which shows the electrical signals generated by the vagus nerve in a mouse injected with a bacterial toxin, is part of the lab’s current effort to better understand the messages the nerve sends to the brain during inflammation. Tracey asks the student to pull up other charts and nerve recordings, and as they chat about the recent findings, an idea for a follow-up experiment occurs to Tracey. He tells the student to begin that very week. Just start with three or four animals, Tracey urges: “What are you waiting for?”
Emily Anthes is a writer in New York City.